To continue our discussion of the somatic mutation theory of cancer (SMT) vs. the mitochondrial metabolic theory (MMT), we here review the scientific literature concerning additional experiments or studies that challenge the foundation of the SMT.
Non-Mutagenic Origin of Metastatic Behavior
Recent studies from Brook Chernet and Michael Levin have shown that alterations in bioelectric membrane signaling can produce the metastatic behavior of Xenopus melanocytes in the absence of somatic mutations, which further suggests that the tumorigenic phenotype is not dependent on nuclear gene mutations (43,44). In other words, nuclear gene mutations alone are insufficient for producing tumors, whereas the tumorigenic phenotype can be produced in some cells without nuclear mutations.
The Absence of Gene Mutations in Cancer Cells
The SMT is based on the premise that nuclear gene mutations are responsible for the dysregulated division of cancer cells. Stuart Baker, however, has reviewed gene sequencing studies that failed to detect any pathogenic somatic mutations in several types of cancers (45). Michael Kiebish et al. also found no pathogenic mutations in mitochondrial DNA from five independently derived mouse brain tumors (46). Moreover, Donald Williams Parsons et al. could find no mutations in any of the three signaling systems of sample Br20P that was obtained from aggressive glioblastoma (47).
Cancer Driver Genes Found in Normal Cells
Recent studies show that normal cells in various tissues contain cancer driver genes. Inigo Martincorena and his team recently found large numbers of cancer driver genes in normal human skin (48,49). Indeed, the prevalence of NOTCH1 mutations in normal esophagus tissue was several times higher than in esophageal cancers. Somatic driver mutations found in brain tissue have been linked to psychiatric disorders rather than to cancer (50). These findings are concerning given that new immunotherapies could target driver mutations in normal cells as well as in tumor cells (48). Significant adverse effects involving inadvertent targeting of normal tissue cells have already been seen in some patients treated with immunotherapies (51).
The Cancer Drug Temozolomide Increases Driver Mutations in Brain Tumors
The toxic alkylating agent Temozolomide (TMZ) is the most common chemotherapy drug used for managing malignant brain tumors and produces a slight increase in progression-free survival for patients (52). Recent studies show that TMZ increases driver mutations in the brain tumor tissue of treated patients (53). How is it possible that a drug, which increases driver mutations, could also improve progression-free survival? The findings make no sense in light of the SMT but could be linked to the MMT. For example, some of the adverse effects of TMZ include fatigue, nausea, vomiting, diarrhea, and loss of appetite (54). All of these adverse effects are indirect forms of calorie restriction that would lower blood glucose levels, thus targeting the Warburg effect and the dependency of tumors on glucose.
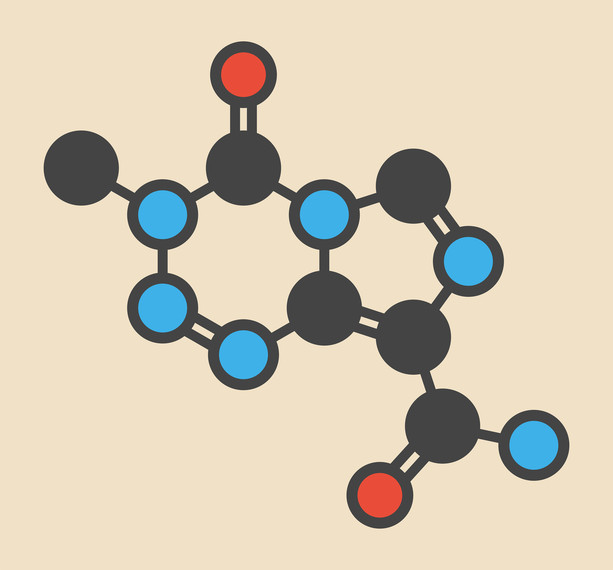
Temozolomide molecule
The Fallacy of Tumor Cell Growth Advantage
According to the SMT, the mutations in tumor cells confer a growth advantage over normal cells. These mutations were thought to produce cells with greater fitness and adaptability to survive in hostile microenvironments than normal cells. This comparison between cancer cells and normal cells makes no sense, as differentiated normal cells are programmed to divide only when needed. However, if normal cells need to grow, they can grow faster than most tumor cells. For example, the growth rate of regenerating liver cells is faster than that of most tumor cells (17).
Tumor cells survive in hypoxic environments not because they are more fit than normal cells, but because they have lost their ability to respire and rely on the ancient pathway of fermentation to generate energy. This should not be considered an advantage but rather a pathological condition. The fermentation metabolism together with defective respiration produces oxygen radicals that damage the genome. Cells with a damaged genome are generally less fit than cells with an intact genome.
In addition to glucose fermentation, tumor cells also can generate energy from amino acid fermentation. Besides excessively consuming glucose, tumor cells consume excessive amounts of glutamine. Glutamine is the most abundant amino acid in the blood and is abundantly present in the microenvironment of most tumors. Glutamine generates energy through mitochondrial substrate-level phosphorylation. This is another ancient metabolic pathway for energy generation in hypoxic environments.
In the final post in this series, we will consider the totality of evidence indicating that the mitochondrial metabolic theory may explain the facts of cancer better than the somatic mutation theory.
Related
- Is Cancer a Genetic or Metabolic Disease? Part 1
- Is Cancer a Genetic or Metabolic Disease? Part 2
- Is Cancer a Genetic or Metabolic Disease? Part 3
- Is Cancer a Genetic or Metabolic Disease? Part 4
- Is Cancer a Genetic or Metabolic Disease? Part 6
Thomas N. Seyfried is professor of biology at Boston College. He received a doctorate in genetics and biochemistry from the University of Illinois—Urbana-Champaign in 1976. He did his undergraduate work at the University of New England, where he recently received the distinguished Alumni Achievement Award. He also holds a master’s degree in genetics from Illinois State University. Seyfried served with distinction in the United States Army’s 1st Cavalry Division during the Vietnam War and received numerous medals and commendations.
He was a postdoctoral fellow in the Department of Neurology at the Yale University School of Medicine and then served on the faculty as an assistant professor in neurology. Seyfried previously served as chair of the Scientific Advisory Committee for the National Tay-Sachs and Allied Diseases Association. He recently received a Lifetime Achievement Award from the Academy of Complementary and Integrative Medicine and the Uncompromising Science Award from the American College of Nutrition for his work on cancer.
He presently serves on several editorial boards, including those for Nutrition & Metabolism, Neurochemical Research, the Journal of Lipid Research, and ASN Neuro. Seyfried has over 180 peer-reviewed publications and is author of the book “Cancer as a Metabolic Disease: On the Origin, Management, and Prevention of Cancer” (Wiley Press).
References
Note: These references include those previously published in “Is Cancer a Genetic or Metabolic Disease? Part 1,” “Part 2,” “Part 3” and “Part 4.”
- Siegel RL, Miller KD, and Jemal A. Cancer statistics, 2018. CA: A Cancer Journal for Clinicians 68.1(2018): 7-30. Available here.
- Hanahan D and Weinberg RA. Hallmarks of cancer: the next generation. Cell 144.5(2011): 646-674. Available here.
- Vogelstein B, Papadopoulos N, Velculescu VE et al. Cancer genome landscapes. Science 339.6127(2013): 1546-1558. Available here.
- Hou JP and Ma J. DawnRank: discovering personalized driver genes in cancer. Genome Medicine 6.7(2014): 56. Available here.
- Iranzo J, Martincorena I, and Koonin EV. Cancer-mutation network and the number and specificity of driver mutations. Proceedings of the National Academy of Sciences of the United States of America 115.26(2018): E6010-E6019. Available here.
- Fearon ER and Vogelstein B. A genetic model for colorectal tumorigenesis. Cell 61.5(1990): 759-767. Available here.
- Tomasetti C and Vogelstein B. Variation in cancer risk among tissues can be explained by the number of stem cell divisions. Science 347.6217(2015): 78-81. Available here.
- Vaux DL. In defense of the somatic mutation theory of cancer. BioEssays 33.5(2011): 341-343. Available here.
- McLeod HL. Cancer pharmacogenomics: early promise, but concerted effort needed. Science 339.6127(2013): 1563-1566. Available here.
- Ju J, Zhu A, and Yuan P. Progress in targeted therapy for breast cancer. Chronic Diseases and Translational Medicine 4.3(2018): 164-175. Available here.
- Seyfried TN. Cancer as a Metabolic Disease: On the Origin, Management and Prevention of Cancer. Hoboken, New Jersey: John Wiley & Sons, Inc., 2012. Available here.
- John AP. Dysfunctional mitochondria, not oxygen insufficiency, cause cancer cells to produce inordinate amounts of lactic acid: the impact of this on the treatment of cancer. Medical Hypotheses 57.4(2001): 429-431. Available here.
- Kim A. Mitochondria in cancer energy metabolism: culprits or bystanders? Toxicological Research 31.4(2015): 323-330. Available here.
- Pelicano H, Zhang W, Liu J et al. Mitochondrial dysfunction in some triple-negative breast cancer cell lines: role of mTOR pathway and therapeutic potential. Breast Cancer Research 16.5(2014): 434. Available here.
- Srinivasan S, Guha M, Dong DW et al. Disruption of cytochrome c oxidase function induces the Warburg effect and metabolic reprogramming. Oncogene 35.12(2016): 1585-1595. Available here.
- Stefano GB and Kream RM. Cancer: mitochondrial origins. Medical Science Monitor 21(2015): 3736-3739. Available here.
- Warburg O. On the origin of cancer cells. Science 123.3191(1956): 309-314. Available here.
- Chinopoulos C and Seyfried TN. Mitochondrial substrate-level phosphorylation as energy source for glioblastoma: review and hypothesis. ASN Neuro 10(2018): 1-27. Available here.
- Boveri T. Concerning the origin of malignant tumours by Theodor Boveri. Translated and annotated by Henry Harris. Journal of Cell Science 121. Suppl.1(2008): 1-84. Available here.
- Nowell PC. The clonal evolution of tumor cell populations. Science 194.4260(1976): 23-28. Available here.
- Weinberg RA. The Biology of Cancer. New York City, New York: Garland Science, 2007. Available here.
- Darlington CD. The plasmagene theory of the origin of cancer. British Journal of Cancer 2.2(1948): 118-126. Available here.
- Rous, P. Surmise and fact on the nature of cancer. Nature 183(1959): 1357-1361. Available here.
- Baker SG and Kramer BS. Paradoxes in carcinogenesis: new opportunities for research directions. BMC Cancer 7(2007): 151. Available here.
- Soto AM and Sonnenschein C. The somatic mutation theory of cancer: growing problems with the paradigm? Bioessays 26.10(2004): 1097-1107. Available here.
- Sonnenschein C and Soto AM. Somatic mutation theory of carcinogenesis: why it should be dropped and replaced. Molecular Carcinogenesis 29.4(2000): 205-211. Available here.
- Burgio E and Migliore L. Towards a systemic paradigm in carcinogenesis: linking epigenetics and genetics. Molecular Biology Reports 42.4(2015): 777-790. Available here.
- Seyfried TN. Cancer as a mitochondrial metabolic disease. Frontiers in Cell and Developmental Biology 3(2015): 43. Available here.
- Li L, Connelly MC, Wetmore C et al. Mouse embryos cloned from brain tumors. Cancer Research 63.11(2003): 2733-2736. Available here.
- McKinnell RG, Deggins BA and Labat DD. Transplantation of pluripotential nuclei from triploid frog tumors. Science 165.3891(1969): 394-396. Available here.
- Hochedlinger K, Blelloch R, Brennan C et al. Reprogramming of a melanoma genome by nuclear transplantation. Genes & Development 18.15(2004): 1875-1885. Available here.
- McNutt M. Journals unite for reproducibility. Science 346.6210(2014): 679. Available here.
- Baker M. 1,500 scientists lift the lid on reproducibility. Nature 533.7604(2016): 452-454. Available here.
- Nosek BA and Errington TM. Making sense of replications. eLife 6: e23383, 2017. Available here.
- Sonnenschein C and Soto AM. Theories of carcinogenesis: an emerging perspective. Seminars in Cancer Biology 18.5(2008): 372-377. Available here.
- Fidler IJ. The pathogenesis of cancer metastasis: the ‘seed and soil’ hypothesis revisited. Nature Reviews Cancer 3.6(2003): 453-458. Available here.
- Tarin D. Cell and tissue interactions in carcinogenesis and metastasis and their clinical significance. Seminars in Cancer Biology 21.2(2011): 72-82. Available here.
- Garvin S, Oda H, Arnesson LG et al. Tumor cell expression of CD163 is associated to postoperative radiotherapy and poor prognosis in patients with breast cancer treated with breast-conserving surgery. Journal of Cancer Research and Clinical Oncology 144.7(2018): 1253-1263. Available here.
- Gast CE, Silk AD, Zarour L et al. Cell fusion potentiates tumor heterogeneity and reveals circulating hybrid cells that correlate with stage and survival. Science Advances 4.9(2018): eaat7828. Available here.
- Pawelek JM and Chakraborty AK. The cancer cell–leukocyte fusion theory of metastasis. Advances in Cancer Research 101(2008): 397-444. Available here.
- Seyfried TN and Huysentruyt LC. On the origin of cancer metastasis. Critical Reviews in Oncogenesis 18.1-2(2013): 43-73. Available here.
- Pawelek JM and Chakraborty AK. Fusion of tumour cells with bone marrow-derived cells: a unifying explanation for metastasis. Nature Reviews Cancer 8.5(2008): 377-386. Available here.
- Chernet BT, Fields C and Levin M. Long-range gap junctional signaling controls oncogene-mediated tumorigenesis in Xenopus laevis embryos. Frontiers in Physiology 5(2015): 519. Available here.
- Chernet BT and Levin M. Transmembrane voltage potential of somatic cells controls oncogene-mediated tumorigenesis at long-range. Oncotarget 5.10(2014): 3287-3306. Available here.
- Baker SG. A cancer theory kerfuffle can lead to new lines of research. JNCI Journal of the National Cancer Institute 107.2(2015): dju405. Available here.
- Kiebish MA and Seyfried TN. Absence of pathogenic mitochondrial DNA mutations in mouse brain tumors. BMC Cancer 5(2005): 102. Available here.
- Parsons DW, Jones S, Zhang X et al. An integrated genomic analysis of human glioblastoma multiforme. Science 321.5897(2008): 1807-1812. Available here.
- Martincorena I and Campbell PJ. Somatic mutation in cancer and normal cells. Science 349.6255(2015): 1483-1489. Available here.
- Martincorena I, Fowler JC, Wabik A et al. Somatic mutant clones colonize the human esophagus with age. Science 362.6417(2018): 911-917. Available here.
- Nishioka M, Bundo M, Iwamoto K et al. Somatic mutations in the human brain: implications for psychiatric research. Molecular Psychiatry 2018. Available here.
- Gauci ML, Boudou P, Baroudjian B et al. Occurrence of type 1 and type 2 diabetes in patients treated with immunotherapy (anti- PD-1 and/or anti-CTLA-4) for metastatic melanoma: a retrospective study. Cancer Immunology, Immunotherapy 67.8(2018): 1197-1208. Available here.
- Stupp R, Hegi ME, Mason WP et al. Effects of radiotherapy with concomitant and adjuvant temozolomide versus radiotherapy alone on survival in glioblastoma in a randomised phase III study: 5-year analysis of the EORTC-NCIC trial. The Lancet Oncology 10.5(2009): 459-466. Available here.
- Johnson BE, Mazor T, Hong C et al. Mutational analysis reveals the origin and therapy-driven evolution of recurrent glioma. Science 343.6167(2014): 189-193. Available here.
- Seyfried TN, Flores R, Poff AM et al. Metabolic therapy: a new paradigm for managing malignant brain cancer. Cancer Letters 356. 2 Pt. A(2015): 289-300. Available here.
- Seyfried TN, Yu G, Maroon JC and D’Agostino DP. Press-pulse: a novel therapeutic strategy for the metabolic management of cancer. Nutrition and Metabolism 14.19(2017). Available here.
All links accessed Feb. 12, 2019.
Comments on Is Cancer a Genetic or Metabolic Disease? Part 5
Thank you for these great articles. Are there thoughts on what causes the tumor cell to lose its ability to respire normally to begin with? thank you
Aleksandra if you go back to the first part it says : "The MMT argues that cancer arises primarily from defects in energy production through oxidative phosphorylation (OxPhos) in the mitochondria. OxPhos generates the majority of energy for most cells of the body. Defects in the number, structure, and function of mitochondria will cause cells to gradually replace insufficient respiration with fermentation for energy production, thus initiating the path to neoplasia. Aerobic fermentation of lactic acid, also called the Warburg effect, is recognized as the most common pathological phenotype of cancer."
Thank you for all the information thus far!
Is Cancer a Genetic or Metabolic Disease? Part 5
3