In this influential 2009 paper, Matthew Vander Heiden, Lewis Cantley, and Craig Thompson provide an alternative explanation for the Warburg effect. The authors argue that cancer cells rely heavily on aerobic glycolysis, not for reasons related to energy requirements, but rather to create the substrates for rapid cell proliferation.
As background: A glucose molecule can be broken down through three processes relevant to this discussion. Oxidative phosphorylation is the primary process normal cells use in the presence of oxygen and delivers 36 molecules of ATP for each molecule of glucose consumed. When a normal cell lacks oxygen, it is forced to use anaerobic glycolysis, which breaks glucose down into lactate and only generates two molecules of ATP for each molecule of glucose. Cancer cells instead use aerobic glycolysis, breaking glucose down to lactate even when oxygen is present and delivering four molecules of ATP for each molecule of glucose consumed.
Vander Heiden et al. first note an analogy between cancer cells and unicellular organisms. While unicellular organisms are in an environment with abundant nutrients, they gear metabolism toward processes that generate biomass, ethanol, and organic acids to encourage proliferation; conversely, when energy is scarce, they seek to maximize the energy extracted from available nutrients. In multicellular organisms, uncontrolled proliferation generally is suppressed, so cells maximize energy extraction rather than biomass production. Cancer cells, on the other hand, take in and use nutrients like a unicellular organism in a proliferative state.
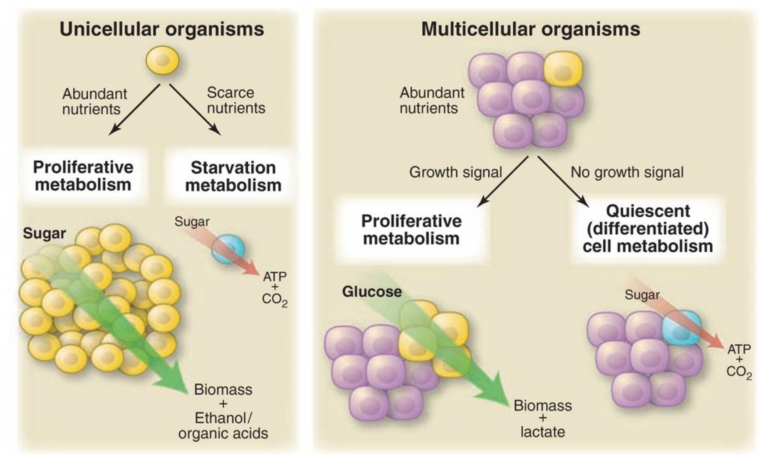
Figure 1, Microbes and cells from multicellular organisms have similar metabolic phenotypes under similar environmental conditions. Unicellular organisms undergoing exponential growth often grow by fermentation of glucose into a small organic molecule such as ethanol. These organisms, and proliferating cells in a multicellular organism, both metabolize glucose primarily through glycolysis, excreting large amounts of carbon in the form of ethanol, lactate, or another organic acid such as acetate or butyrate. Unicellular organisms starved of nutrients rely primarily on oxidative metabolism, as do cells in a multicellular organism that are not stimulated to proliferate. This evolutionary conservation suggests that there is an advantage to oxidative metabolism during nutrient limitation and nonoxidative metabolism during cell proliferation. From Understanding the Warburg Effect,The Metabolic Requirements of Cell Proliferation.
In 1924, Otto Warburg noted that cancer cells, unlike normal tissues, ferment glucose into lactate even when there is sufficient oxygen to support oxidative phosphorylation (i.e., they perform aerobic glycolysis). In other words, cancer cells metabolize glucose, using pathways that generate less energy (ATP) per glucose molecule consumed despite having the resources necessary to use a more efficient process. While the observation is widely recognized, there is no recognized explanation for why cancer cells behave this way.
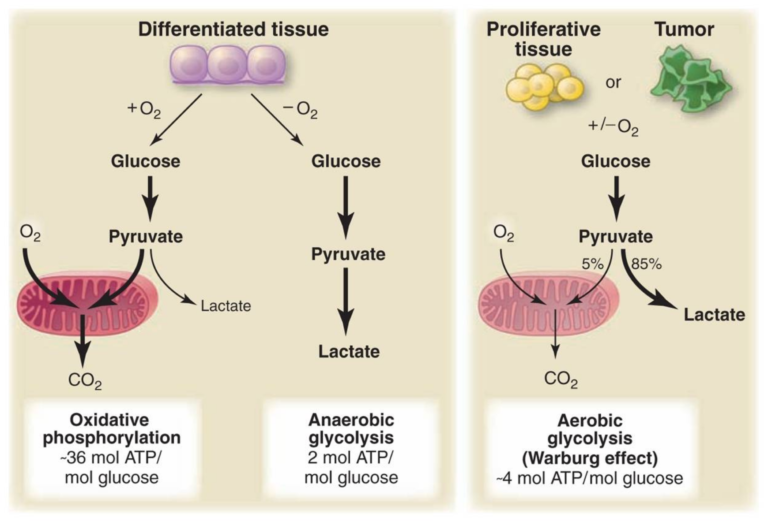
Figure 2, Schematic representation of the differences between oxidative phosphorylation, anaerobic glycolysis, and aerobic glycolysis (Warburg effect). In the presence of oxygen, nonproliferating (differentiated) tissues first metabolize glucose to pyruvate via glycolysis and then completely oxidize most of that pyruvate in the mitochondria to CO2 during the process of oxidative phosphorylation. Because oxygen is required as the final electron acceptor to completely oxidize the glucose, oxygen is essential for this process. When oxygen is limiting, cells can redirect the pyruvate generated by glycolysis away from mitochondrial oxidative phosphorylation by generating lactate (anaerobic glycolysis). This generation of lactate during anaerobic glycolysis allows glycolysis to continue (by cycling NADH back to NAD+), but results in minimal ATP production when compared with oxidative phosphorylation. Warburg observed that cancer cells tend to convert most glucose to lactate regardless of whether oxygen is present (aerobic glycolysis). This property is shared by normal proliferative tissues. Mitochondria remain functional and some oxidative phosphorylation continues in both cancer cells and normal proliferating cells. Nevertheless, aerobic glycolysis is less efficient than oxidative phosphorylation for generating ATP. In proliferating cells, ~10% of the glucose is diverted into biosynthetic pathways upstream of pyruvate production. From Understanding the Warburg Effect,The Metabolic Requirements of Cell Proliferation.
The rapid growth of cancer cells (and closer mechanistic studies) demonstrates that their use of aerobic glycolysis does not prevent them from generating sufficient energy to survive and reproduce. Instead, the authors argue, the more pressing constraint on proliferation may be the production of the nucleotides, amino acids, and lipids necessary to replicate the entire contents of the cell before mitosis. While production of these raw constituents of growth, such as palmitate and amino acids, require some ATP, their requirements for carbon and other substrates are greater relative to what glucose can provide.
As an example of these requirements: “to make a 16-carbon fatty acyl chain, a single glucose molecule can provide five times the ATP required, whereas 7 glucose molecules are needed to generate the NADPH required.” In other words, if the majority of glucose is committed to ATP production, the cell will have insufficient substrate to rapidly replicate its contents and proliferate. This is true for a variety of molecules, as glucose and glutamine “supply most of the carbon, nitrogen, free energy and reducing equivalents necessary to support cell growth and division.”
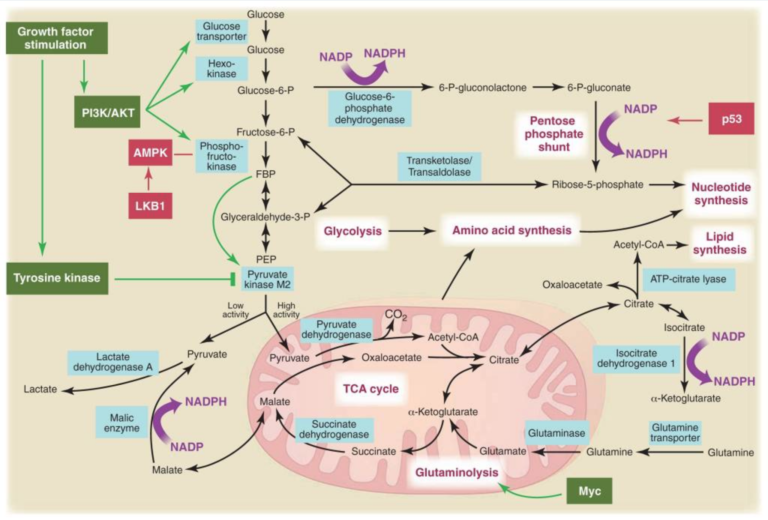
Figure 3, Metabolic pathways active in proliferating cells are directly controlled by signaling pathways involving known oncogenes and tumor suppressor genes. This schematic shows our current understanding of how glycolysis, oxidative phosphorylation, the pentose phosphate pathway, and glutamine metabolism are interconnected in proliferating cells. This metabolic wiring allows for both NADPH production and acetyl-CoA flux to the cytosol for lipid synthesis. Key steps in these metabolic pathways can be influenced by signaling pathways known to be important for cell proliferation. Activation of growth factor receptors leads to both tyrosine kinase signaling and PI3K activation. Via AKT, PI3K activation stimulates glucose uptake and flux through the early part of glycolysis. Tyrosine kinase signaling negatively regulates flux through the late steps of glycolysis, making glycolytic intermediates available for macromolecular synthesis as well as supporting NADPH production. Myc drives glutamine metabolism, which also supports NADPH production. LKB1/AMPK signaling and p53 decrease metabolic flux through glycolysis in response to cell stress. Decreased glycolytic flux in response to LKB/AMPK or p53 may be an adaptive response to shut off proliferative metabolism during periods of low energy availability or oxidative stress. Tumor suppressors are shown in red, and oncogenes are in green. Key metabolic pathways are labeled in purple with white boxes, and the enzymes controlling critical steps in these pathways are shown in blue. Some of these enzymes are candidates as novel therapeutic targets in cancer. Malic enzyme refers to NADP+-specific malate dehydrogenase [systematic name (S)-malate:NADP+ oxidoreductase (oxaloacetate-decarboxylating)]. From Understanding the Warburg Effect,The Metabolic Requirements of Cell Proliferation.
Thus, the authors posit, glucose and glutamine metabolism in a proliferating cancer cell is designed to maximize production of cellular building blocks rather than energy production. The proliferative state is thus “turned on” by a simultaneous increase in nutrient acquisition by the tumor cells and a shift toward metabolic pathways that support proliferation.
Comments on Understanding the Warburg Effect: The Metabolic Requirements of Cell Proliferation
I am writing to address issues regarding the role of the Warburg effect in cancer that was presented in the linked Vander Heiden et al paper. Much of the confusion regarding the Warburg effect has been clarified since the paper was first published in Science 10 years ago.
Vander Heiden et al correctly argue that cancer cells rely heavily on aerobic glycolysis (Warburg effect), not for reasons related to energy requirements, but rather to create the substrates for rapid cell proliferation. The authors also indicate that mitochondrial OxPhos must be normal in cancer cells if aerobic glycolysis is not the source of ATP synthesis. They go on to state “while the Warburg effect is widely recognized, there is no recognized explanation for why cancer cells behave this way”.
The recent paper by Chinopoulos and Seyfried has now clarified much of the confusion surrounding the Warburg effect (ASN Neuro, DOI: 10.1177/1759091418818261). Cancer cells continue to ferment lactic acid in the presence of oxygen because they have abnormalities in the number, structure, and function of their mitochondria. Whether ATP is generated through glycolysis depends largely on the pyruvate kinase (PK) isoform present in the tumor cells. ATP synthesis is greater in cells with the PKM1 isoform than in cells with the PKM2 isoform. Interestingly, many cancer cells express the PKM2 isoform thus making ATP synthesis through glycolysis limited. Chinopoulos and Seyfried reviewed extensive evidence showing that significant ATP synthesis could also be generated through mitochondrial substrate level phosphorylation (mSLP) in cancer cells that express both the PKM2 isoform and have abnormal mitochondria. In other words, ATP synthesis through mSLP can compensate for reduced ATP synthesis in tumor cells with abnormal mitochondria and the PKM2 isoform.
This phenomenon was described as the “Warburg Q-effect”, as glutamine (Q) becomes the major fermentable substrate through the glutaminolysis pathway (glutamine---glutamate---alpha-ketoglutarate---succinyl CoA---succinate). In addition to glucose, it is well known that glutamine is also a major fuel for cancer cells. Besides replenishing metabolites in the TCA cycle (anaplerosis), glutamine can also generate significant ATP through the succinyl CoA synthase reaction. Vander Heiden et al appeared unaware of the role of glutamine in generating energy through mSLP.
Both the Warburg effect and the Warburg Q-effect arise from compromised OxPhos. Hence, Recognition of mSLP as a second major compensatory energy mechanism for tumor cells with defective or insufficient OxPhos will have profound implications for managing most tumors including GBM.
The Warburg Q-effect is now recognized as the missing link in Otto Warburg’s central theory that cancer arises initially from environmental or genetic damage to a cell’s mitochondrial OxPhos. ATP synthesis through substrate level phosphorylation in the cytoplasm (glycolysis) or through substrate level phosphorylation in the mitochondria (mSLP) would gradually compensate for the reduced ATP synthesis from OxPhos. This process then leads to loss of growth control, genomic mutations, and abnormal proliferation, i.e., neoplasia.
Another misconception from the Vander Heiden et al paper was that aerobic glycolysis is required not only for cancer cell proliferation but also for proliferation of non-transformed cells grown in culture, thus giving the impression that aerobic glycolysis is a normal phenomenon of cell proliferation. This phenomenon does not occur in proliferating non-transformed cells grown in vivo. For example, a number of investigators showed that regenerating liver cells and normal colon cells use fatty acids and butyrate as fuel, respectively. Mike Kiebish et al showed that the cell culture growth environment suppressed Complex I activity and forced normal astrocytes to ferment through a Crabtree-linked effect (doi:10.1042/AN20090011). This cell culture effect would obscure differences in energy metabolism between normal cells and cancer cells. Indeed, Warburg and Burk cautioned against generalizations made on cellular energy metabolism based solely on studies from cell culture environments. As Chinopoulos and Seyfried described, respiratory impairment or inhibition, arising from either genetic or environmental causes, will force cells into a fermentation metabolism with consequent proliferation. The formation of lipid droplets, as is seen in many cancer cells, is further evidence of respiratory impairment. Lipids should be used as a respiratory fuel in cells with normal respiration capacity, and not stored as droplets in the cytoplasm. This new information has improved our understanding of the Warburg effect and the metabolic abnormalities that underlie cell proliferation in normal cells and in cancer cells.
Thanks for taking the time to clarify.
Understanding the Warburg Effect: The Metabolic Requirements of Cell Proliferation
2